Our plants are hugely affected by carbon dioxide levels in our atmosphere. Green plants absorb CO2 to produce sugars for growth (and oxygen for us!) in a process known as photosynthesis, meaning CO2 levels can hugely affect plant function.
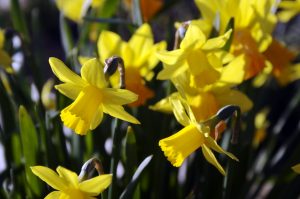
CO2 levels are currently at 406 parts per million (ppm), which may not seem high, but have risen 21% over the last 230 years (Woodward, 1987). This fluctuated constantly throughout time, varying from 280 to 370ppm over the last 24 million years (Van Der Burgh et al, 1993), but now for the first time exceeds 400ppm (Khatiwala et al, 2009).
CO2, oxygen and water are absorbed and released through small pores on the underside of leaves known as stomata, protected by guard cells which open and close the pore. This is affected by conditions such as hormones, light, and- you guessed it- atmospheric CO2 concentration. Stomata allow plants to survive stress, for example retaining water during extreme heat and drought (Hetherington & Woodward, 2003).
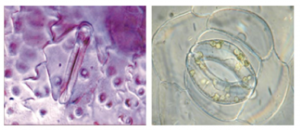
How do we investigate change in stomata over time? We can find out what plants were like thousands of years ago- that’s where the Egyptian King Tutankhamun comes in. Olive leaves from his tomb (from 1327 BC) were taken, and the amount of stomata on the underside counted and compared to Egyptian olive samples from 332BC, 1818AD, 1978AD and 1991AD (Beerling & Chaloner, 1993a).
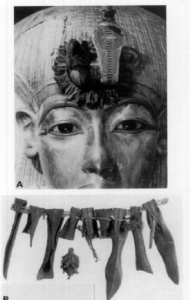
As CO2 increases, the number of stomata decrease (Beerling & Chaloner, 1993a). This is also seen in other species, such as oak (Van der Burgh et al, 1993) and pine (Van de Water et al, 2007), but their methods weren’t as creative! The first ever study to show this change found a 40% decrease in number of stomata over the last 230 years (Woodward, 1987).
Why does this happen? CO2 affects the genetic make-up of plants, reducing the number of cells developing into stomata. Plants are likely adapting to rising temperatures and CO2 levels by decreasing the amount of pores to reduce water loss, improving their water use efficiency (Beerling & Chaloner, 1993b).
Is this change a problem for plants? The short term effects of more CO2 can be beneficial, increasing photosynthesis and growth and therefore yield (Osborne et al, 1997), however the long term effects are not so good. Fewer stomata decreases CO2 uptake, reducing growth and leading to higher atmospheric CO2 (Reddy et al, 2004). Although the plants retain more water, if CO2 levels and temperature decrease again, plants with fewer stomata will have reduced water use efficiency (Woodward, 1987) as they will not be able to exchange as well. Less sugar production also reduces metabolism, therefore CO2 intake is inhibited even further (Flexas & Medrano, 2002) in a cycle known as negative feedback; a reduction in one factor causes a further reduction in something else.
This shows us that plants have changed greatly over thousands of years to adapt to the increasing carbon dioxide levels around them, which could have long-term negative effects on both plants and other life. The environment around us is changing with the atmosphere, and human-caused CO2 increase should not be ignored as it affects many things, no matter how small.
Word Count: 469
References
Beerling, D. J., Chaloner, W. G. 1993 a. Stomatal Density Responses of Egyptian Olea europaea L. Leaves to CO2 Change to 1327BC. Annals of Botany, 71: 431-435
Beerling, D. J., Chaloner, W. G. 1993 b. Evolutionary Responses of Stomatal Density to Global CO2 Change. Biological Journal of the Linnean Society, 48: 343-353
Flexas, J., Medrano, H. 2002. Drought Inhibition of Photosynthesis in C3 Plants: Stomatal and non-Stomatal Limitations Revised. Annals of Botany, 89(2): 183-189
Hetherington, A. M., Woodward, F. I. 2003. The Role of Stomata in Sensing and Driving Environmental Change. Nature, 424: 901-908
Khatiwala, S., Primeau, F., Hall, T. 2009. Reconstruction of the History of Anthropogenic CO2 Concentrations in the Ocean. Nature, 462: 346-350
Osborne, C. P., Drake, B. G., LaRoche, J., Long, S. P. 1997. Does Long Term Elevation of CO2 Concentration Increase Photosynthesis in Forest Floor Vegetation? (Indiana Strawberry in a Maryland Forest). Plant Physiology, 114(1): 337-344
Reddy, A. R., Chaitanya, K. V., Vivekanandan, M. 2004. Drought-Induced Responses of Photosynthesis and Antioxidant Metabolism in Higher Plants. Journal of Plant Physiology, 161(11): 1189-1202
Van Der Burgh, J., Visscher, H., Dilcher, D., Kürschner, W. M. 1993. Paleoatmospheric Signatures in Neogene Fossil Leaves. Science, 260(5115): 1788-1790
Van de Water, P. K., Leavitt, S. W., Betancourt, J. L. 2007. Trends in Stomatal Density and 13C/12C Ratios of Pinus flexilis Needles During the last Glacial-Interglacial Cycle. Science, 264: 239-243
Woodward, F. I. 1987. Stomatal Numbers are Sensitive to Increases in CO2 from pre-Industrial Levels. Nature, 327: 617-618
Recent Comments