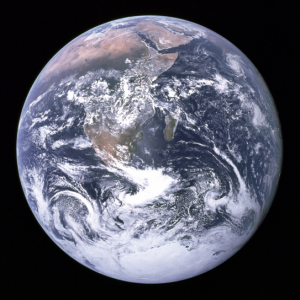
In 1972, one of the most iconic photographs of the Earth was taken from space. The ‘Blue Marble’ snapped by the astronauts aboard Apollo 17 shows an Earth with deep blue oceans but very little greenery on the land. Now photographs of the Earth from space look very different, with luscious green patches where there was once dull brown. The spreading and growing of green vegetation is a result of rising CO2 levels in the Earth’s atmosphere. The Earth has undergone an increase of 18 square kilometres of new vegetation between 1982 and 2009 (Keenan et al., 2016).
Since the Industrial Revolution, the burning of fossil fuels such as coal and oil by humans has caused an enormous rise in atmospheric CO2 from 280ppm to over 400ppm today, inducing disastrous effects on the environment such as climate change (Khatiwala et al., 2009).
So if increases in CO2 are so bad, why is a boom in plant growth occurring?
Plants use CO2 in photosynthesis; a process in which plants use CO2, water and light from the sun to produce sugars for growth and oxygen which they give off. The increased rates of photosynthesis are down to a chemical called Rubisco, which helps incorporate CO2 into the photosynthesis process. Rubisco first evolved long, long ago- far before humans began affecting the world. At this point in history CO2 levels were much greater than during recent times. This means that Rubisco is less efficient at lower CO2 levels. As humans have begun to disturb these lower CO2 concentrations and caused them to rise, Rubisco works better meaning plants are able to photosynthesise at a greater rate, which increases their growth (Taylor et al., 1994).
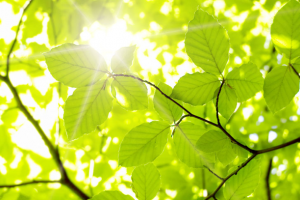
Although this appears to be all good news for the plants, rising atmospheric CO2 levels also bring negative effects, one of them being climate change. With increasing CO2 comes increases in temperature which can negatively impact plants. Plants require an optimum temperature in order to survive well and if they are not able to shift their ranges, they will suffer the effects of warmer, dryer environments which are ultimately inhospitable (Hatfield and Prueger, 2015). So whilst plants prosper in the short term, when temperatures get too high they languish.
Also, there is evidence that rising CO2 reduces the ability of stomata- small pores on plants- to conduct CO2 and perform transpiration- the removal of water-, ultimately leading to reduced photosynthesis (Drake et al., 1997).
Although rising CO2 has turned the brown swathes of Earth captured in the ‘Blue Marble’ into luscious green, behind this initial bloom lurks an ominous truth. If humans continue to fuel rising CO2 levels, plants will suffer and food crops will fail. Global temperature increases, rainfall changes and extreme weather events- droughts and floods- jeopardise the functions of plants, ultimately devastating them.
Word Count: 468
References
Drake, B.G., Gonzalez-Meler, M.A., Long, S.P.,1997. More efficient plants: a consequence of rising atmospheric CO? Annu. Rev. Plant Physiol. Plant Mol. Biol. 48, 609 – 639.
Hatfield, J.L. and Prueger, J.H., 2015. Temperature extremes: effect on plant growth and development. Weather and Climate Extremes, 10, pp.4-10.
Keenan, T.F., Prentice, I.C., Canadell, J.G., Williams, C.A., Wang, H., Raupach, M. and Collatz, G.J., 2016. Recent pause in the growth rate of atmospheric CO2 due to enhanced terrestrial carbon uptake. Nature communications, 7.
Khatiwala, S., Primeau, F. and Hall, T., 2009. Reconstruction of the history of anthropogenic CO2 concentrations in the ocean. Nature, 462(7271), pp.346-349.
NASA/ Apollo 17 Crew., 1972., ‘Blue Marble’ –Earth as seen by Apollo 17. [Photograph]
Shuttershock., 2013., Sun through leaves. [Photograph]
Taylor, G., Ranasinghe, S., Bosac, C., Gardner, S.D.L. and Ferris, R., 1994. Elevated CO2 and plant growth: cellular mechanisms and responses of whole plants. Journal of Experimental Botany, 45(Special Issue), pp.1761-1774.
Recent Comments